By Nirmal John
Quantum Computing: The Revolutionary Technology Shaping Our Future
Thursday April 3, 2025
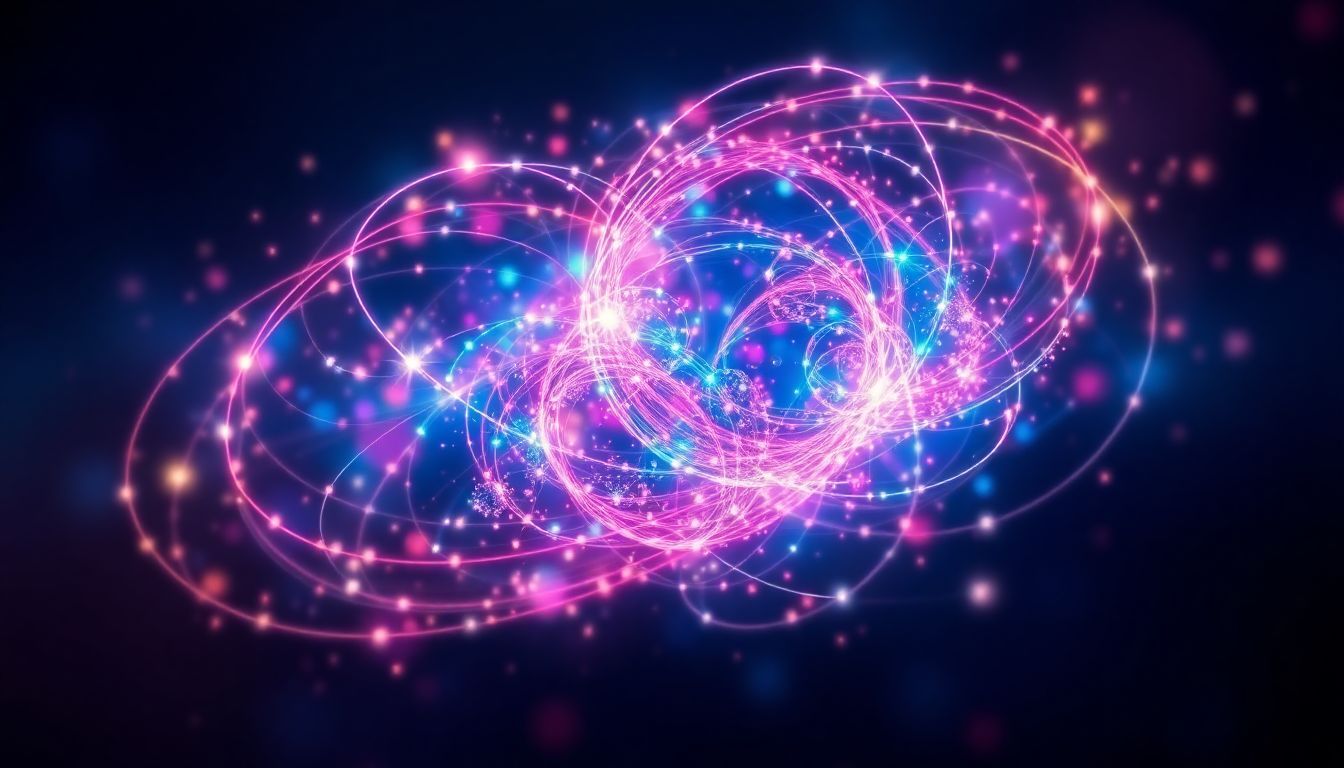
Quantum Computing: The Revolutionary Technology Shaping Our Future
Introduction to Quantum Computing’s Transformative Power
In the landscape of technological evolution, quantum computing represents not just an advancement but a fundamental paradigm shift. Imagine trading a horse-drawn carriage for a rocket ship—that’s the magnitude of change we’re experiencing with quantum computing technology. Traditional computers, despite their remarkable capabilities, are increasingly hitting performance walls when faced with complex computational problems. Quantum computers, however, offer a revolutionary approach to break through these limitations.
The emergence of quantum computing is poised to redefine multiple industries by solving problems that have long been considered computationally intractable. From drug discovery to climate modeling, financial optimization to artificial intelligence—the potential applications extend far beyond what we can currently envision. This disruptive technology isn’t just a theoretical concept anymore; it’s a developing reality that forward-thinking organizations are already beginning to harness.
As we stand at this technological crossroads, understanding quantum computing becomes increasingly essential for businesses, researchers, and technology enthusiasts alike. This comprehensive guide explores the fundamental principles, current capabilities, and future potential of quantum computing in an accessible yet detailed manner.
Understanding Quantum Computing: Core Principles
What is Quantum Computing?
Quantum computing represents a fundamentally different approach to computation that leverages the principles of quantum mechanics to process information. Unlike classical computers that operate using bits (binary digits representing either 0 or 1), quantum computers utilize quantum bits or “qubits.” The revolutionary aspect of qubits lies in their ability to exist in multiple states simultaneously, thanks to a quantum phenomenon called superposition.
This distinctive capability allows quantum computers to perform certain types of calculations at speeds unattainable by even the most powerful supercomputers. While classical computers process information sequentially, quantum computers can explore multiple computational paths concurrently. This parallelism gives quantum computing its extraordinary potential to tackle problems that would otherwise remain unsolvable using traditional computing approaches.
The field has progressed significantly in recent years, moving from theoretical concepts to working prototypes and early commercial systems. Companies like IBM, Google, and Microsoft have developed quantum processors with increasingly higher qubit counts, pushing the boundaries of what’s computationally possible.
Key Quantum Principles: Superposition and Entanglement
To truly grasp the transformative potential of quantum computing, one must understand its two foundational principles: superposition and entanglement.
Superposition can be conceptualized through the famous thought experiment of Schrödinger’s cat, which exists in a state of being both alive and dead simultaneously until observed. Similarly, a qubit in superposition exists as both 0 and 1 until measured, creating a computational space that grows exponentially with each additional qubit. This property enables quantum computers to work with vast amounts of potential solutions simultaneously.
Entanglement, perhaps even more remarkable, creates a connection between qubits that transcends conventional physical limitations. When qubits become entangled, the state of one instantaneously affects the state of another, regardless of the distance separating them. Einstein famously referred to this as “spooky action at a distance.” In quantum computing, entanglement serves as a powerful resource that allows for the creation of highly correlated quantum states, essential for the implementation of complex quantum algorithms.
Together, these quantum properties create computational capabilities that extend far beyond classical limitations, particularly for specific classes of problems like factoring large numbers, database searching, and simulating quantum systems.
Qubit Technologies: Diverse Approaches to Quantum Processing
The development of stable, scalable qubits represents one of the most significant challenges in quantum computing. Currently, researchers are pursuing several different technological approaches, each with distinct advantages and limitations:
Superconducting qubits have gained significant traction among major companies like IBM and Google. These qubits consist of tiny electrical circuits cooled to near absolute zero temperatures, where quantum effects begin to dominate. Superconducting technology offers manufacturing scalability using modified semiconductor fabrication techniques, but requires extensive cooling infrastructure and faces coherence time limitations.
Trapped ion qubits, championed by companies like IonQ and Honeywell, use individual charged atoms suspended in electromagnetic fields as their quantum bits. These systems boast superior coherence times and qubit fidelity but present challenges in scaling to large numbers of qubits.
Photonic quantum computing leverages the quantum properties of light particles. This approach offers potential advantages in room-temperature operation and networking capabilities but faces challenges in creating reliable two-qubit gates.
Other emerging approaches include topological qubits, which theoretically offer error protection at the hardware level, and neutral atom qubits, which position atoms in optical lattices to perform quantum operations.
The diversity of these approaches highlights that quantum computing remains in its exploratory phase, with different architectures potentially proving optimal for different applications as the technology matures.
Quantum Computing vs. Classical Computing: A Comparative Analysis
Processing Power and Speed: The Quantum Advantage
When comparing quantum computing with classical computing, the differences in processing capabilities become most apparent in specific problem domains. Quantum computers don’t simply offer incremental improvements in speed—they can potentially solve certain problems exponentially faster than their classical counterparts.
One of the most frequently cited examples is integer factorization, a process that underpins much of modern cryptography. Using Shor’s algorithm, a quantum computer could theoretically factor large numbers in polynomial time, a task that would require exponential time on classical computers. This particular capability has significant implications for cybersecurity, potentially rendering current encryption methods vulnerable.
The concept of “quantum supremacy” (sometimes called “quantum advantage”) represents a milestone where a quantum computer performs a specific calculation that would be practically impossible for any classical computer. Google claimed to have achieved this milestone in 2019 with their 53-qubit Sycamore processor, completing a specialized calculation in 200 seconds that would allegedly take the world’s most powerful supercomputer thousands of years.
However, it’s crucial to understand that quantum computers won’t replace classical computers for general-purpose computing. Rather, they will serve as specialized accelerators for specific computational tasks where quantum algorithms offer substantial advantages.
Algorithm Complexity and Limitations: Understanding the Trade-offs
Despite their remarkable potential, quantum computing systems face significant practical challenges. One of the most pressing is quantum decoherence—the tendency of quantum systems to lose their quantum properties when interacting with their environment. This phenomenon leads to computational errors and limits the time available to perform calculations before the quantum information degrades.
Quantum error correction represents a vital area of research addressing this challenge. Unlike classical error correction, quantum error correction must preserve the delicate quantum states without directly measuring them (which would collapse the superposition). Current approaches require multiple physical qubits to create a single error-protected logical qubit, significantly increasing the hardware requirements for practical quantum computers.
Additionally, developing quantum algorithms requires specialized expertise in quantum mechanics and computer science. The counterintuitive nature of quantum mechanics makes algorithm development particularly challenging. Currently, only a limited set of quantum algorithms have been discovered that offer proven advantages over classical approaches.
Furthermore, the programming models for quantum computers differ substantially from classical computing paradigms, creating a steep learning curve for developers entering the field. Organizations like IBM Quantum are working to develop more accessible programming frameworks, but the field remains technically demanding.
The Hybrid Approach: Leveraging Classical and Quantum Systems Together
The most practical implementation of quantum computing in the near and medium term will likely involve hybrid systems that combine quantum and classical computing elements. This approach recognizes the complementary strengths of both paradigms and leverages each where most appropriate.
In a typical hybrid quantum-classical architecture, classical computers handle pre-processing of data, problem formulation, and post-processing of results, while quantum processors tackle the computationally intensive portions of algorithms where they offer advantages. This collaborative approach is already being implemented in early quantum cloud services offered by companies such as Amazon Web Services Braket.
Quantum-inspired algorithms represent another interesting development in this hybrid ecosystem. These are classical algorithms that incorporate insights from quantum computing theory to achieve performance improvements, even without using actual quantum hardware. Microsoft has been particularly active in developing such approaches for optimization problems.
As quantum technology continues to mature, the boundary between quantum and classical computing will likely become increasingly fluid, with sophisticated orchestration systems determining the optimal processing approach for different computational tasks within complex workflows.
Real-World Applications of Quantum Computing
Drug Discovery and Materials Science: Accelerating Innovation
One of the most promising applications of quantum computing lies in simulating molecular and chemical systems. Classical computers struggle with accurately modeling the quantum behavior of molecules, particularly as their complexity increases. Quantum computers, however, are naturally suited for simulating quantum systems, potentially revolutionizing fields like pharmaceutical research and materials science.
In drug discovery, quantum computers could model the interactions between drug candidates and biological targets with unprecedented accuracy. This capability could significantly accelerate the identification of promising compounds, reducing the time and cost of bringing new treatments to market. Companies like Boehringer Ingelheim are already partnering with quantum computing providers to explore these possibilities.
For materials science, quantum simulation could enable the design of new materials with precisely tailored properties—from more efficient solar cells to better batteries and superconductors. The ability to accurately predict material properties before synthesis could transform manufacturing processes across multiple industries.
These applications are particularly exciting because they align well with the near-term capabilities of quantum computers. Even with limited qubit counts, useful simulations of specific molecular systems may be possible, offering value before fully error-corrected quantum computers become available.
Financial Modeling and Risk Analysis: Transforming Investment Strategies
The financial services industry faces numerous computationally intensive challenges that quantum computing could potentially address. Portfolio optimization, risk assessment, fraud detection, and options pricing all involve complex calculations that could benefit from quantum approaches.
Monte Carlo simulations, widely used in financial risk analysis, represent a particularly promising application area. Quantum algorithms could potentially perform these simulations quadratically faster than classical methods, allowing for more sophisticated risk models and better-informed investment decisions.
Several major financial institutions, including JPMorgan Chase and Goldman Sachs, have established quantum computing research teams to explore these possibilities. While practical implementations may still be years away, the potential competitive advantage is driving significant investment in the field.
Quantum machine learning algorithms also show promise for detecting patterns in financial data that might elude classical analysis methods. These could lead to more effective fraud detection systems and trading strategies based on subtle market signals.
Optimization and Logistics: Solving Complex Operational Challenges
Optimization problems pervade modern business operations, from supply chain management to resource allocation and transportation routing. Many of these problems fall into the category of “combinatorial optimization,” where the goal is to find the best solution from an extremely large set of possibilities.
Quantum computing offers potential advantages for such problems through algorithms like the Quantum Approximate Optimization Algorithm (QAOA) and quantum annealing approaches. These methods could potentially find optimal or near-optimal solutions more efficiently than classical techniques for problems with complex constraints and objectives.
For example, delivery companies could optimize vehicle routing across thousands of destinations, manufacturing firms could improve production scheduling, and airlines could enhance flight planning—all leading to significant cost savings and efficiency improvements.
Companies like Volkswagen have already conducted pilot projects using quantum computing for traffic optimization, demonstrating the practical potential of these approaches even with current quantum hardware limitations.
The Future of Quantum Computing: Challenges and Opportunities
Challenges and Roadblocks: The Path to Practical Quantum Computing
Despite the rapid progress in quantum computing over the past decade, several significant challenges must be overcome before the technology reaches its full potential:
The most fundamental challenge involves creating and maintaining stable qubits. Current quantum systems suffer from high error rates due to decoherence, limiting the complexity of problems they can address. Developing effective quantum error correction techniques and increasing qubit coherence times remain active areas of research.
Scalability presents another major hurdle. While research labs have demonstrated systems with dozens of qubits, scaling to the thousands or millions of qubits needed for many practical applications requires solving complex engineering challenges related to control electronics, cooling systems, and qubit connectivity.
Additionally, the quantum software stack remains relatively immature. Developing programming languages, compilers, and debugging tools that abstract away quantum complexities while preserving quantum advantages requires significant research investment.
Standardization efforts are also just beginning, with organizations like the IEEE working to establish common frameworks and benchmarks for quantum computing systems. This standardization will be crucial for the technology’s commercial adoption.
Investment and Research Landscape: Growing Momentum
Despite these challenges, investment in quantum computing continues to accelerate. Major technology companies have established substantial quantum research programs. IBM has made significant investments in its IBM Quantum program, offering cloud access to quantum processors. Google, Microsoft, Amazon, and Intel have all established major quantum initiatives as well.
The startup ecosystem is equally active, with companies like Rigetti Computing, IonQ, and PsiQuantum attracting substantial venture funding. These startups are pursuing diverse technological approaches and application-specific solutions.
Government funding worldwide has also increased dramatically, with the United States, China, the European Union, and others launching multi-billion dollar national quantum initiatives. These programs recognize the strategic importance of quantum technology for economic competitiveness and national security.
Research partnerships between industry, academia, and government laboratories have become increasingly common, accelerating the transfer of theoretical advances to practical implementations. This collaborative ecosystem is essential for addressing the multidisciplinary challenges of quantum computing development.
Quantum Computing: A Realistic Timeline for Industry Disruption
While predictions about technological development timelines are inherently uncertain, a general consensus is emerging about the likely progression of quantum computing:
In the next 2-3 years, we can expect continued improvements in quantum hardware, with qubit counts and coherence times steadily increasing. Early commercial applications will focus on narrow use cases where even noisy, intermediate-scale quantum (NISQ) devices can provide value.
Over the 5-7 year horizon, error correction techniques will likely mature, enabling more reliable quantum computation. This period may see the first clear quantum advantages in commercially relevant applications, particularly in chemistry simulation and specific optimization problems.
Looking 10-15 years ahead, fault-tolerant quantum computers with thousands of logical qubits may become available, potentially enabling transformative applications in cryptography, machine learning, and material design.
Industries should prepare for this progression by building quantum literacy within their organizations, identifying potential application areas relevant to their business, and establishing partnerships with quantum technology providers. Those who position themselves early in this technological revolution will likely gain significant competitive advantages as the technology matures.
Conclusion: Preparing for the Quantum Future
Quantum computing represents one of the most profound technological shifts on the horizon. Its development will likely follow the pattern of many transformative technologies—progressing through periods of enthusiastic hype, temporary disillusionment, and eventually, practical implementation and widespread adoption.
The potential benefits across industries are enormous. From discovering life-saving drugs to optimizing global logistics networks, creating advanced materials to transforming financial markets, quantum computing promises capabilities that extend far beyond current computational limits.
For organizations and individuals alike, now is the time to begin building quantum awareness and exploring potential applications. Understanding the fundamental principles of quantum computing, staying informed about technological developments, and identifying relevant use cases will be crucial for leveraging this technology as it matures.
Educational resources on quantum computing are becoming increasingly accessible, with online courses, certification programs, and simulation tools allowing for practical exploration without physical quantum hardware.
As we stand at the threshold of the quantum era, those who prepare will be best positioned to harness its transformative potential. Quantum computing is no longer a distant future—it’s an emerging reality that will reshape industries and create new possibilities we’re only beginning to imagine.
Resources for Further Learning
- Quantum Computing Report – Industry news and analysis
- Qiskit – Open-source quantum computing software development framework
- National Quantum Initiative – U.S. government quantum information resource
- Quantum Computing for the Very Curious – Accessible introduction to quantum computing concepts
About the author
Recent articles
Quantum Computing: The Revolutionary Technology Shaping Our Future
Quantum Computing: The Revolutionary Technology Shaping Our Future Introduction to Quantum Computing’s Transformative Power In...
Read MoreQuad Core Dedicated Servers
Interested in Quad Core Dedicated Servers? View our inventory. What is a quad core dedicated...
Read More8 Core Dedicated Servers
For website owners looking to eliminate their hardware bottlenecks, the massive power capabilities of an...
Read More